Recently, the research team led by associate professor Lijie Zhou of the School of the College of Chemistry has published a paper entitled Non-electroactive bacteria behave variously in AnMBR biofilm control using electric field in Water Research, a leading environmental engineering journal recognized in the Nature Index, JCR Chemistry Region 1, TOP Journal. The team's associate professor Lijie Zhou is the primary author and corresponding author. Shenzhen University is the first author unit.
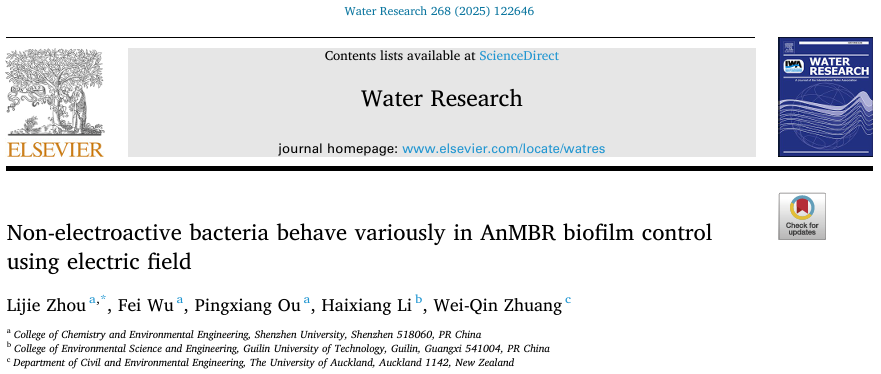
Electric fields have been widely applied in anaerobic membrane bioreactors (AnMBRs). Another notable application of electric fields is to directly provide electrons for bacterial degradation metabolism, such as in microbial electrolysis cells (MECs). These electroactive bacteria can directly harvest electrons from solid electrodes and transfer electrons between each other through conductive appendages like nanowires and pili. Consequently, electroactive bacteria typically utilize an external electric field to enrich soluble electron donors without the need for any electric field. Compared to the significant focus on electroactive bacteria, non-electroactive bacteria, which cannot directly transfer electrons from electrodes to electrodes or vice versa, have seldom been exploited by electrochemical techniques. Despite this limitation, recent evidence suggests that non-electroactive bacteria, which dominate the bacterial kingdom, may play some significant roles in electrofield-mediated processes. For instance, in low-voltage electric field bioreactors predominantly containing non-electroactive bacteria, most of the bacterial cells within the biofilm appear to be non-electroactive. Therefore, we are interested in whether non-electroactive bacteria are significantly affected by electric fields. During the operation of the E-AnMBR, what role do non-electroactive bacteria play in controlling biofilm formation through electric field control?
In this study, we investigated the behavior of non-electroactive bacteria in the E-AnMBR, focusing on their contribution to biofilm formation and their response to various low-voltage electric fields used to control biofilm formation. By examining the community function and functional gene abundance of non-electroactive bacteria, we aim to elucidate the interaction between electricity and non-electroactive bacteria during biofilm formation. Understanding these dynamics will provide new insights into the role of non-electroactive bacteria in biofilm control, broadening the scope of bioelectrochemical applications beyond the traditional focus on electroactive organisms.
Firstly, no current was detected in the four E-AnMBRs with the applied potential, indicating that water electrolysis did not occur during the experimental process. As previously mentioned, some electroactive bacteria are reported to use cytochrome c for electron transfer. However, our analysis of metagenomic-assembled genomes (MAGs) revealed Thauera, Desulfomicrobium, and Pseudomonas, which carry complete or partial denitrification genes but lack cytochrome c-encoding genes, suggesting that these MAGs are not electroactive bacteria. Additionally, infrared scanning of the biofilm samples showed no characteristic absorption wavelength at 1405 cm^-1, which is used to indicate the presence of cytochrome c. Taken together, all this evidence suggests that the copper mesh functioning as the cathode electrode may generate an electromagnetic field that repels bacteria (and other naturally occurring organic matter).
Observation of non-electroactive bacterial biofilm formation through SEM revealed that the biofilm thickness ranged from 350 to 800 μm (Figure S7). Compared to the biofilm in S4 (468 μm), it was found that both too low (0.3 V) and too high (1 V) electric field voltages hindered the growth of non-electroactive bacterial biofilm on the membrane surface. The 0.5 V electric field (S2) significantly increased the thickness of the non-electroactive bacterial biofilm (780 μm), reaching 1.6 times that of S4. Furthermore, the higher absolute zeta potential value of S2 (0.5 V) (31.6 mV, p < 0.01) also supported this (Figure S8). It can be seen that the electric field significantly regulates the development of non-electroactive bacterial biofilm, and the 0.5 V electric field markedly stimulates the formation of non-electroactive bacteria on the membrane surface. Further research found that a lower PN/PS ratio was thought to increase the irreversible biofilm formation of non-electroactive bacteria on the membrane. Figures 2(a) and (b) visually show that the PN/PS values are S1 (1 V) > S3 (0.3 V) > S4 (0 V) > S2 (0.5 V), with S3 (0.3 V) and S1 (1 V) increasing the PN/PS value over S4, while S2 (0.5 V) decreased it. This result is consistent with the observation that the biofilm in S2 is the thickest. Under the action of the 0.5 V electric field, the highest nucleic acid content was found (26.8 mg/g VSS, p < 0.01). At the same time, the thicker the biofilm in S2, the higher its nucleic acid content is likely to be. This result explains why the 0.5 V electric field exacerbates irreversible pollution caused by the formation of non-electroactive bacterial biofilm on the membrane surface. In addition, the higher dehydrogenase activity (DHA) content in S2 (9.36 mg/g VSS, p < 0.01) also supports this conclusion (Figure d). In the biological treatment process, high DHA produces more metabolic waste, leading to more severe biofilm formation by non-electroactive bacteria. Compared with S4, the DHA content in S2 increased, while the DHA content in S3 and S1 was inhibited. Therefore, the 0.5 V electric field can promote the formation of non-electroactive bacterial biofilm and accumulate on the membrane surface.
To make sense of the mechanism involved in this process that a lower voltage electric field caused thicker biofilm in E-AnMBR, the metagenomic technology was used to detect the functional gene abun dance of the non-electroactive bacteria community, so that the meta bolic network diagram in the community was mapped. Profiles of non- electroactive bacteria’s metabolic pathways with electric field were identified from the gene level by metagenomic analysis. The functional genes encoding for enzymes of metabolic pathways with high abun dance were labeled based on the KEGG database (Fig. 3). Compared with S4, the biosynthesis and metabolism genes of various organic substances related to non-electroactive bacterial growth (i.e., carbohydrate meta bolism, lipid metabolism and amino acid metabolism) showed an overall upward trend in S2 (Fig. 3c), while S1 and S3 showed an overall downward trend (Fig. 3a). This result indicated that the electric field stimulated the metabolic capacity of non-electroactive bacteria. Compared with S4, quorum sensing (QS) genes in S2 were much more abundant than those in S1 and S3 (Fig. 3b). The increase in QS genes might be helpful for information exchange between bacteria and the biofilm formation of non-electroactive bacteria (Sun et al. 2018). Electric fields can also interfere with signal pathways and communica tion mechanisms in microbial communities, including quorum sensing, which is very important for the development of biofilm (Zhou et al. 2023b). This was further confirmed by the exuberant metabolic activ ities of the amino acids (i.e., Glyoxylate and dicarboxylate, Pyruvate, and Glycine serine and threonine) (Fig. 3a). The increase of functional genes encoding for enzymes of the fatty acid metabolic pathway indicated a possibility that more acetyl-CoA could be fueled into the citrate cycle (Fig. 4a). It is also confirmed by a high abundance of functional genes encoding for succinate dehydro genase [1.3.5.1], succinyl-CoA synthetase [6.2.1.5] and 2-oxoglutarate reductase [1.2.7.3] in citrate cycle pathway (Fig. 4a). Meanwhile, a high abundance of functional genes encoding for succinate dehydroge nase [1.3.5.1] and 2-oxoglutarate reductase [1.2.7.3] indicated that more FADH 2 and NADH, substrates for oxidative phosphorylation, would be produced. Therefore, the electron transfer speed of oxidative phosphorylation was accelerated. This indicated that FADH 2 and NADH were accumulated, and electron transfer in oxidative phosphorylation was promoted. Indeed, obviously increasing functional genes encoding for enzymes of the oxidative phosphorylation pathway were noticed (Fig. 4b), similar to most other genes encoding for enzymes involved in the citrate cycle (Fig. 4a). Overall, the electric field (at 0.5 V) significantly impacted the biofilm formation of non-electroactive bacteria by regulating carbon metabolism to phosphorylation.
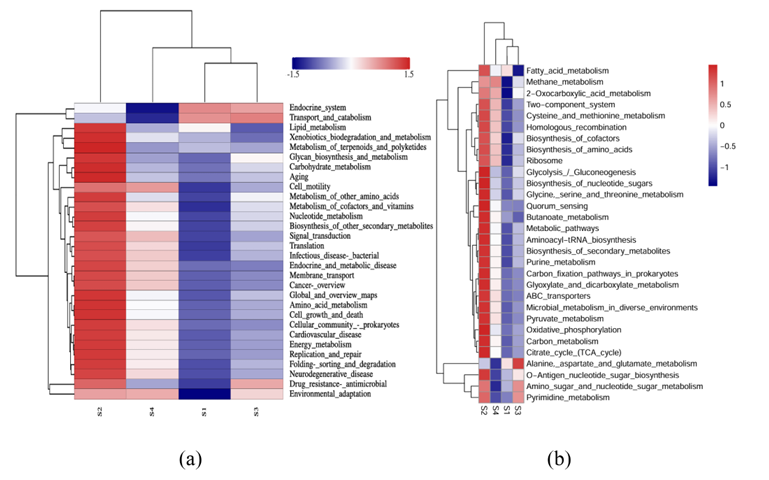
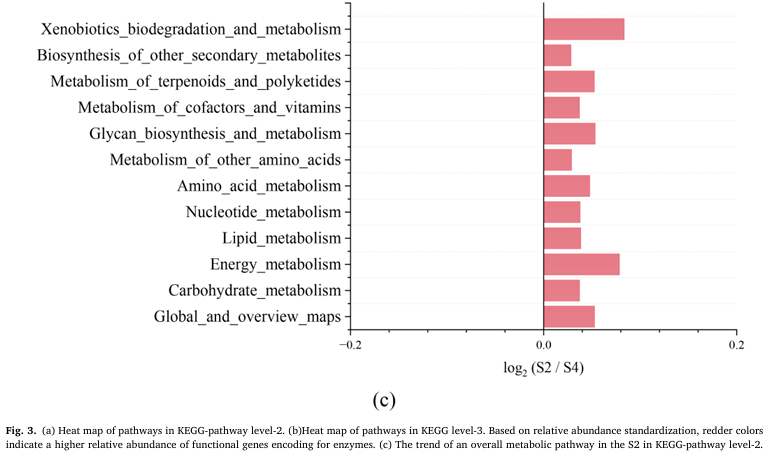
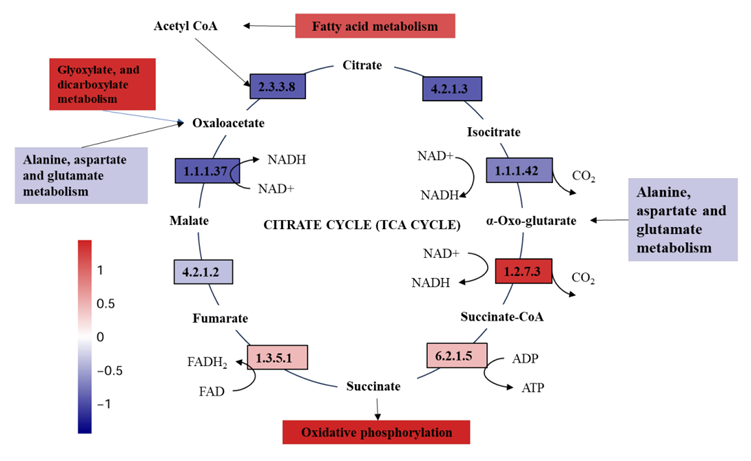
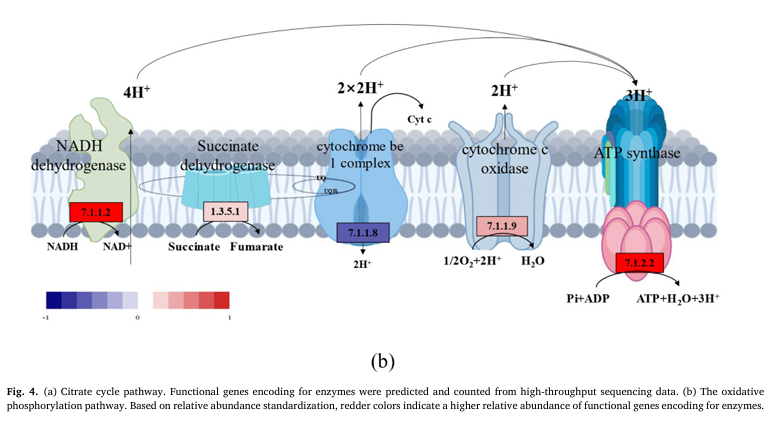
This study was funded by “Guangdong Basic and Applied Basic Research Foundation”, the (Key) Project of Department of Education of Guangdong Province” and “Shenzhen Science and Technology Program”.
See full text link:
https://www.sciencedirect.com/science/article/abs/pii/S0043135424015458